Green ammonia – a sustainable energy source with great potential
Green hydrogen as a sustainable energy carrier is considered trend-setting for the energy transition. However, another substance could become even more important in the near future: green ammonia.
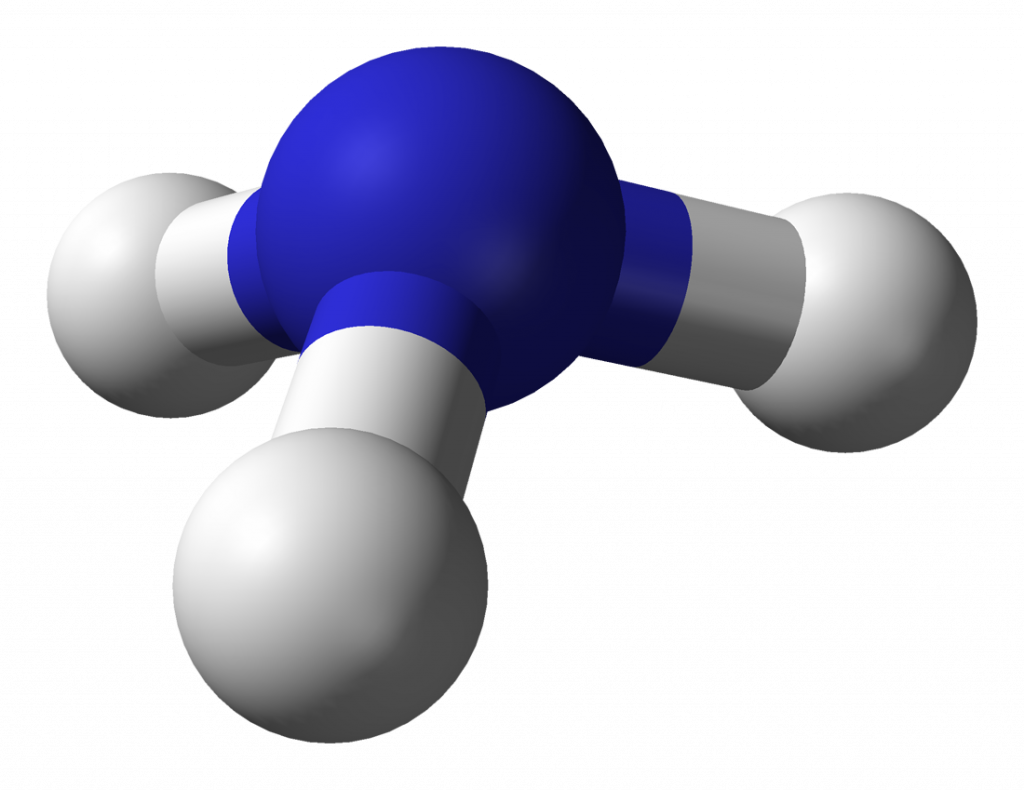
Today, around 80% of the 170 million tons of ammonia produced annually worldwide is used as a basic chemical for fertilizers. Only 20% is used for other technical solutions. However, in the coming years, demand for ammonia will shift drastically towards new markets: power generation and transportation. Ammonia is the cheapest liquid fuel for long-term storage, easily shipped globally, and a viable marine fuel.
While in the past most ammonia plants used natural gas as feedstock, today all the signs point to green ammonia,” explains Thore Lohmann, Executive Director of the Ammonia and Methanol business at thyssenkrupp Uhde. “The increasing installation of applications for renewable energy, reduced electricity costs, the introduction of CO2 taxes and possible subsidies for green ammonia technology are drastically changing today’s market drivers towards green ammonia applications.”
Ammonia is a colorless gas at room temperature. The chemical compound consists of nitrogen and hydrogen with the empirical formula NH3.
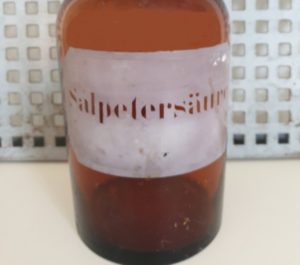
“Green ammonia” describes the manufacturing process: In contrast to ammonia produced conventionally from fossil fuels, green ammonia is based on renewable energies.
Because green ammonia can be used both as a basic chemical, as a fuel and as a comparatively easy-to-transport hydrogen storage medium, the gas plays an important role in the global energy transformation.
According to the experts, one of the most promising applications of green ammonia is as a sustainable energy source. “Ammonia can be produced from the existing elements hydrogen and nitrogen from the air and, if necessary, broken down into its components again with the help of a so-called cracker. For example, ammonia can be shipped from Australia to Japan and used there either directly to generate electricity or for industrial applications be cracked back to hydrogen.”
Ammonia can also be burned directly, e.g. in gas turbines or in ship engines. Due to its flexible application possibilities, ammonia is an ideal green energy molecule. “Compared to hydrogen, ammonia has a higher energy density, which makes it easy to transport and easy to store,” explains Thore Lohmann
Because ammonia is combustible and can also be converted directly into electricity in fuel cells, the chemical is also suitable as an energy carrier. Ammonia burns to form nitrogen and water and is not itself a greenhouse gas. Therefore, ammonia synthesized from green hydrogen is climate-neutral. . In the future, green ammonia could replace climate-damaging bunker oil as ship fuel. According to estimates, the demand for ammonia as clean ship fuel could exceed today’s conventional NH3 production by a factor of six if propulsion systems were to be switched around the world.
This makes green ammonia an ideal liquid energy carrier for transporting “green hydrogen” from renewable energies over long distances. In contrast to pure hydrogen, ammonia has a much higher density, making it easier, more energy-efficient and cheaper to store and transport it than it can. In addition, ammonia is already a globally traded product with existing transport infrastructure and thus offers enormous potential for a global green energy economy and the reduction of greenhouse gas emissions.
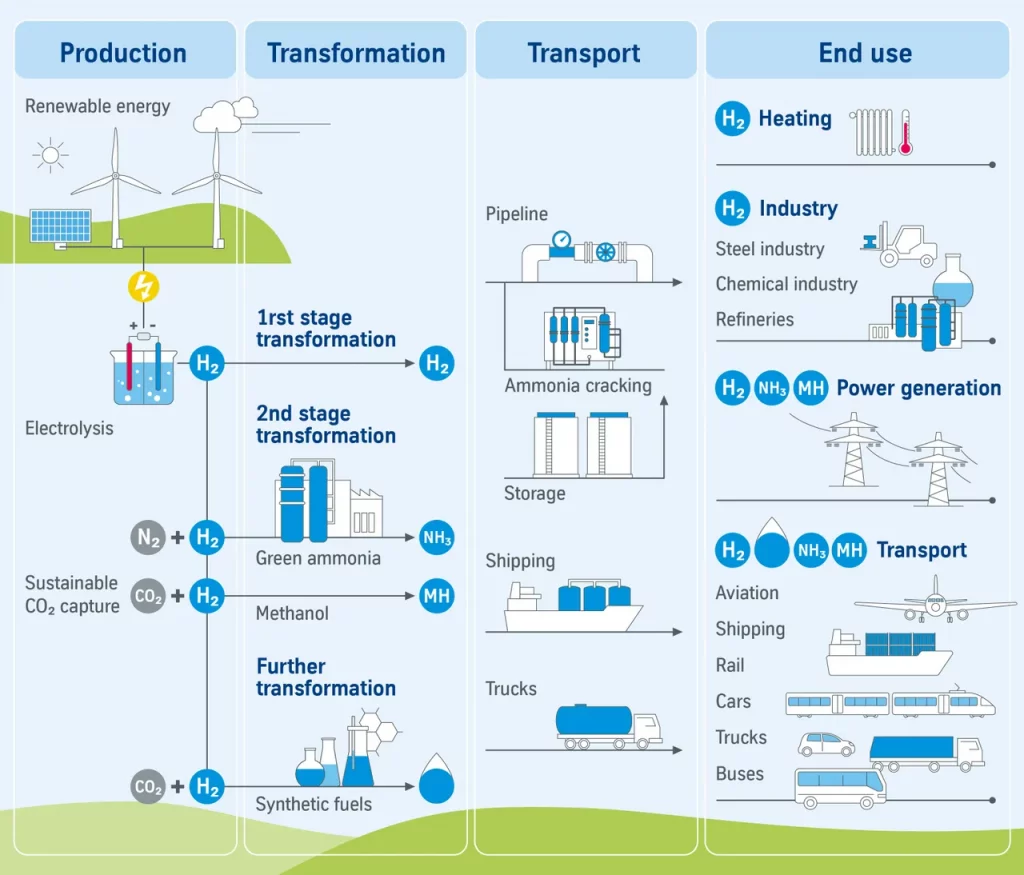
What are the properties of ammonia?
Ammonia has a pungent smell, is colorless and toxic and soluble in water (531 g/l). At -33 °C, the boiling point is significantly higher than that of hydrogen (-253 °C). The vapor pressure is 8.6 bar at 20 °C. The gas is therefore comparatively easy to liquefy, transport and store.
Why green ammonia and not hydrogen?
Green ammonia is produced by catalytic synthesis from separated atmospheric nitrogen and green hydrogen. In the electrolysis of hydrogen from wind or solar power, the efficiency is 70 to 90%, depending on the process. Conversion to ammonia means further losses; the overall efficiency up to reconversion – for example in a steam power plant – is only 55 to 60%. In other words, it would actually be much cheaper to use renewable energies directly or to store them only in the form of hydrogen.
However, the simpler handling, transport and storage of the energy in the form of ammonia speak in favor of this route: Ammonia can be easily transported by ship or pipeline and, due to its low flammability, the risk of explosion is lower than with hydrogen. Since ammonia has a vapor pressure of only 8.6 bar at 20 °C and can be liquefied at -33 °C, the requirements for storage tanks are significantly lower than for hydrogen. At the same time, the energy density of ammonia at ambient temperature is significantly higher than that of hydrogen gas under the same conditions. However, the energy density is only half that of petrol (6.25 kWh/kg) (12.7 kWh/kg). Nevertheless, green ammonia is much easier to handle as a marine fuel than hydrogen.
How is conventional ammonia produced?
The majority of the ammonia produced worldwide (over 90%) is produced in direct synthesis using the Haber-Bosch process. In this reaction, the gases nitrogen and hydrogen react with each other. The nitrogen is obtained by liquefaction of air, the hydrogen required for the reaction by steam reforming from natural gas, coal and naphtha. The catalytic reaction between hydrogen and nitrogen takes place at high pressure. In the conventional production of ammonia from natural gas, 1.5 tons of CO2 are produced per ton of ammonia.
The classic Haber Bosch synthesis: NH3 is now produced on a large scale using the Haber Bosch process, which celebrated its 100th anniversary in 2013. Nitrogen and H2 are passed over an iron catalyst at temperatures of 400 to 500 degrees Celsius and pressures of 150 to 250 bar. These process parameters are a compromise between NH3 thermal stability, reaction rate and catalyst activity. A conversion of 15 percent is achieved under equilibrium conditions. The required N2 is obtained by cryogenic air separation, while H2 is produced by steam reforming of methane CH4.
Two tons of climate-damaging carbon dioxide CO2 are released for every ton of NH3 produced. The valuable hydrocarbons are not converted into the end product NH3, but only into CO2, which cannot yet be used on an industrial scale. Modern Haber Bosch plants produce up to 1500 tons of NH3 per day and consume approximately eight megawatt hours of energy per ton of NH3 based on CH4 and 13.5 megawatt hours of energy based on coal as a hydrogen source.”
How is green ammonia produced?
The production of green ammonia is based on two steps: First, electricity from wind, solar or hydroelectric power is used to electrolyze water. The resulting hydrogen is then catalytically converted with atmospheric nitrogen to form ammonia.
For electrolysis, alkaline electrolysis systems, such as those used for chlorine production, have been in use for many years. Another method is based on so-called proton exchange membranes. The nitrogen required for the synthesis of ammonia is extracted from the air by pressure swing absorption (ASU).
The decentralized production of green ammonia from renewable energies is being investigated in the “Campfire” innovation initiative. In contrast to classic alkali electrolysis or water electrolysis on proton exchange membranes, ceramic thin-film membranes are a central component here. These should achieve a high level of efficiency and service life, making the production processes more economical.
One of the largest plants for green hydrogen is to be built in north-west Saudi Arabia by 2025 (Helios Green Fuels Project). With a 20 MW electrolysis module, 650 tons of hydrogen are to be produced daily on the basis of 4 GW of solar and wind energy and 3,000 tons of ammonia are to be converted. The electrolysis system will be supplied by Thyssenkrupp, and the module manufacturer is being funded by the Federal Ministry of Economics.
Ammonia as a hydrogen vector: New integrated reactor technology for the energy transition
Fraunhofer Institute ISE/University of Ulm:
In the “PICASO” project (Process Intensification & Advanced Catalysis for Ammonia Sustainable Optimized process), the Fraunhofer Institute for Solar Energy Systems ISE, the University of Ulm and the Fukushima Renewable Energy Research Institute (FREA-AIST) are working on a new type of power-to-ammonia – (PtA) process for sustainable ammonia synthesis. The process could reduce CO2 emissions by 95 percent compared to the conventional process. The project, funded by the Federal Ministry of Education and Research, started on August 1, 2022.
Ammonia as a hydrogen vector has the potential to make a significant contribution to the energy transition: “Ammonia can also be produced from green hydrogen and nitrogen in sunny and windy but remote regions – for example in the North African desert. The energy source is liquefied for transport to Europe, usually by ship. To this end, we are developing integrated reactor technology with dynamic operating strategies that allow operation with fluctuating renewable energy sources,” explains Prof. Dr. Christopher Hebling, Head of Hydrogen Technologies at Fraunhofer ISE.
In contrast to the conventional Haber-Bosch process, the PtA process allows the use of more active synthesis catalysts thanks to the high purity of the electrolysis-based “green” hydrogen. These can work at lower temperatures, which increases the thermodynamically possible ammonia yield and thus enables operation at lower pressures and without the recirculation of unused educts. For the project, the Japanese partner FREA-AIST has developed a novel ruthenium catalyst that enables synthesis under significantly milder process conditions with temperatures below 400 °C and pressures below 80 bar. This can already be produced on a semi-industrial scale (TRL > 7). In order to further increase the yield, Fraunhofer ISE and the University of Ulm are investigating the integrated separation of ammonia: The reaction and the separation of ammonia take place in-situ in an integrated reactor. In this way, the operating pressure can be minimized and the recirculation of unreacted feed gas can be avoided. “Since the compressors and heat exchangers are the biggest cost drivers in conventional ammonia synthesis, accounting for 90 percent of the investment costs, these improvements offer enormous potential for the economic efficiency of flexible ammonia production plants that can also be used in remote regions,” says Dr.-Ing. Ouda Salem, group leader Power to Liquids at Fraunhofer ISE. This means that complex infrastructure is no longer necessary and ammonia can be produced on a much smaller scale. This offers the possibility of tailoring the new PtA process for the use of renewable energy sources in remote regions as well. Prof. Dr.-Ing. Robert Güttel, Head of the Institute for Chemical Engineering at the University of Ulm, adds: “In addition, we can make much better use of hydrogen and nitrogen if no recirculation is required, so that we can significantly increase the material and energetic efficiency of the entire PtA process.”
In the project, the transfer of the new PtA concept from the laboratory to the pilot plant scale is already to be implemented. While the focus at the University of Ulm is on the laboratory scale, extensive experimental studies are carried out in the technical center at Fraunhofer ISE. Robert Güttel: “The experimental findings in both scales are linked by detailed mathematical modeling and simulation. With this we can even make reliable predictions for the pilot scale and accelerate the implementation of the integrated reactor concept.” In addition to the technical demonstration, the partners also want to prove that the new, flexible PtA process is economically competitive with the conventional process.
Disruptive process with high savings potential
“If successful, the PICASO approach will be a disruptive technology that replaces a conventional fossil process and thus reduces CO2 emissions by up to 95 percent,” says Ouda Salem. A simulative analysis of the PICASO process also revealed an energy saving potential of 50 percent compared to the conventional Haber-Bosch process. A concrete goal for a follow-up project is the upscaling of the integrated reactor to demonstration level and its testing in a pilot plant at the location of the associated partners FREA-AIST in Fukushima. In addition, the researchers are developing specific dynamic investigations and operational strategies to identify interface requirements between the electrolysers and the synthesis plant. With the successful completion of these project phases, the basic engineering data for an industrial reference system is available. The PICASO partners will accompany these phases with R&D services and own patents on catalyst and reactor developments in order to license the complete technology to the chemical and process engineering industry.
Conclusion
It should be undisputed that green hydrogen and in particular green ammonia will be important energy sources for a future energy transition. The procedural realization seems to be promising, especially as far as the ammonia synthesis according to PICASO is concerned, here there seems to be great progress in terms of sustainability and costs. However, I am critical of the planned locations, especially with regard to security of supply. It makes little sense to look one-sidedly at the advantages of sunny locations such as the Arabian region or North Africa without considering that you are becoming dependent again, which, according to recent experience, should actually be avoided. In particular, the Helios Green Fuels Project in Saudi Arabia raises major question marks with regard to security of supply. Not only is the green hydrogen obtained here, but also the entire ammonia synthesis is carried out on an industrial scale with all the geopolitical imponderables of these areas. Added to this are the long transport routes. As so many promising developments in the field of sustainable climate-friendly technologies ultimately cannot avoid relying primarily on domestic wind and solar energy – or at least investing in Europe – the promising Power to Ammonia projects should not ignore these aspects.
Bernd Riebe, Nov.2022
https://www.thyssenkrupp.com/de/stories/nachhaltigkeit-und-klimaschutz/gruenes-ammoniak-und-sein-beitrag-zur-bewaeltigung-des-klimawandels
https://www.chemietechnik.de/anlagenbau/was-ist-gruenes-ammoniak-123.html
Fraunhofer Institut ISE/Universität Ulm
Deutscher Bundestag: Fachbereich Umwelt, Naturschutz, Reaktorsicherheit, Bildung und Forschung .(Wissenschaftliche Dienste Dokumentation WD 8 – 3000 – 088/18)
Schreibe einen Kommentar